Research.
Research Topic
Multi-Functional Nano-Science of Advanced Metal Complexes
[1] Haldane Gap Compounds
[2] Single-Moleucle Magnets (SMMs)
[3] Single-Chain Magnets (SCMs)
[4] Quantum Molecular Spintroncs Based on Single-Moleucle Magnets
[5] Spin Qubits for Quantum Computer
[6] SMMs Encapsulated into Single Walled Carbon Nano Tube (SWCNT)
[7] Nano-Wire MX-Chain Compounds with Strong Electron-Correlation
[8] Charge Fluctuation in MX-Chain Complexes
Project 1.
Quantum Molecular Spintronics Based on Single-Molecule Magnets: Super Highly Density Memory Device and Molecular Spin Qubits for Quantum Computer
Abstract
Material design mainly focusing on semiconductors has supported various industries, such as electronic components and recording media. Moreover, it is about to be replaced by spintronics which will become the most important materials of the 21st century in the near future. This is a research field that combines the spin of electrons and the electric charge to create a new function. In addition, it is expected to become the ultimate green IT because of fast signal response speed, high density, and low power consumption. However, due to the Moore’s Law, which describes material design centering on classical magnets and silicon semiconductors, the emergence of a new approach to these materials is desired. In this research, we propose a concept of single-molecule magnet (SMM) type spintronics instead of traditional classical magnet type materials for the development of nano-sized magnets. However, insufficient knowledge about function development, etc. make this research difficult. In this research project, we will overcome the problems by closely linking molecular design, synthesis, analysis, function, and theory.
(1) Background of the Research Project
Since organic molecules can maintain their spin currents longer than the metals can, attention is focused on molecular spintronics. The goal is to develop a sustainable and cheap spintronic device using molecular materials. In addition, the long-term goals involve the miniaturization (nanoscale) and the creation of a nano-spintronic device driven by one or a few molecules. However, it is not easy to inject aligned spins (spin current) into organic molecular materials. The cause is the source of the spin current, and the spin-orbit interaction for efficient control is very small. Graphene represented by molecular spintronics utilizes spin-orbit interactions, which are increased by hybridization of π-d orbitals occurring at the interface with a magnetic metal (Ni) electrode. Recently, such spin current control

Fig. 1 SMM based spintronics.
at the interface at the molecular level has been called spinterface (spin + interface). Therefore, in this research project, by using single-molecule magnets (SMMs), which are the new magnets of the 21st century (next-generation type magnet) for spintronics. The spin of nano-sized magnets will bring about new technology for function development (Fig. 1). SMMs are completely different from inorganic magnets, and it is possible to control their electronic states, ligand fields, and magnetic anisotropies by combining various metal ions in different electronic states and organic ligands. For example, cobalt (Co) nanoparticles, which are classical magnets (ferromagnetic metals), decrease the energy required for reversal of the magnetization, and behave as paramagnets (superparamagnetic material). With the spontaneous reversal of the magnetization, the nanoparticles stop operating as magnetic memory. On the other hand, since the barrier energy for magnetization reversal (Ueff) is formed inside the molecule by strong uniaxial magnetic anisotropy, SMMs behave as magnets even on the nanoscale (Fig. 2).
(2) Research Objectives and Targeted Goals of Project
Fig. 2 SMM has strong uniaxial anisotropy which forms a double-well potential.
Therefore, since SMM functions as a magnetic memory with one molecule, the recording density will dramatically increase. Meanwhile, rare earth (f electron) SMMs, which are of particular interest in this project, exhibit spin-orbit interactions, meaning conversion among magnetization, a current signal, and spin current are possible. In other words, SMMs can be used to control the spin current more easily than organic molecular materials can. However, to the best of our knowledge, there have been no reports on spin injection into SMMs. Therefore, the applicant aims to create functional spintronics using SMMs and to create new fields of study in spintronics, such as (1) technology development enabling spin injection/detection from a ferromagnetic electrode to SMM, (2) demonstration of
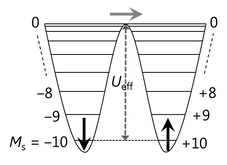
Fig. 2 SMM has strong uniaxial anisotropy which forms a double-well potential.
single spin addressing and reading, (3) spin-dependent transport phenomenon of SMMs, (4) design of spintronic devices using a multifunctional molecular spin system, (5) improvement of the function as a spin qubit; and so on. In particular, for SMMs, controlling the spin at the atomic/nano level and express spin transport characteristics is important because, in advanced technology, not only synthesis but also the development of measurement methods is required. Therefore, the main challenge of this research is to prepare SMM-based spin devices. Currently, the low-power consuming semiconductors used for small equipment (smartphones, digital cameras, memory, and CPUs) are complementary metal-oxide semiconductors (CMOSs). However, by combining molecular nanomagnets/SMMs and low-energy consumption information processing based on spintronic effects, the applicant expects to achieve further miniaturization, weight reduction, and new functions. Therefore, it is possible to deal with actual scientific and technical problems by utilizing molecular spin materials.
(3) Research Plan and Method
In this research project, we propose the concept of single-molecule magnet (SMM) type spintronics to replace conventional classical magnet materials and create new technology via the development of the functions of spinning nano-sized magnets. The research contents (a)–(e) are explained below (Fig. 3).
(a) Efficient synthesis method for high-performance SMMs for nano-spintronic devices and functional evaluation (Fig. 3a).
We will synthesize SMMs with electrochemical/optical switching functions and clarify their functionality by using magnetic measurement and spectroscopic techniques.
(b) Development of operation and control methods of spins in SMMs (Fig. 3b).
For the operation and control of spins in SMMs, we aim to detect the spin direction (↑ · ↓) by performing conductance analysis using spin-polarized tunneling current via STM/STS. The magnetization state of one SMM molecule is detected by the current, and from the measurement of the spin-polarized current, it can be verified if an SMM can magnetically record information. Moreover, its mechanism can be clarified.
(c) Understanding and clarification of the spin-dependent transport phenomenon of conductive SMMs (Fig. 3c).
As part of the spin-dependent transport phenomenon of conductive SMM, we will clarify the effect of π-f interactions between localized f spins and conduction carriers and f ion dependence. The SMM is placed on the gate portion of a FET device, the current between the source and the drain is controlled in the state of the spin, and the mechanism is clarified.
(d) Development of quantum bit manipulation and control methods utilizing self-assembly of SMMs (Fig. 3d).
We aim to lengthen the coherence time by utilizing the self-assembly of SMMs and clarify the correlation with molecular aggregation morphology and dimensionality. We possess pulse ESR and can conduct detailed research on the coherence time.
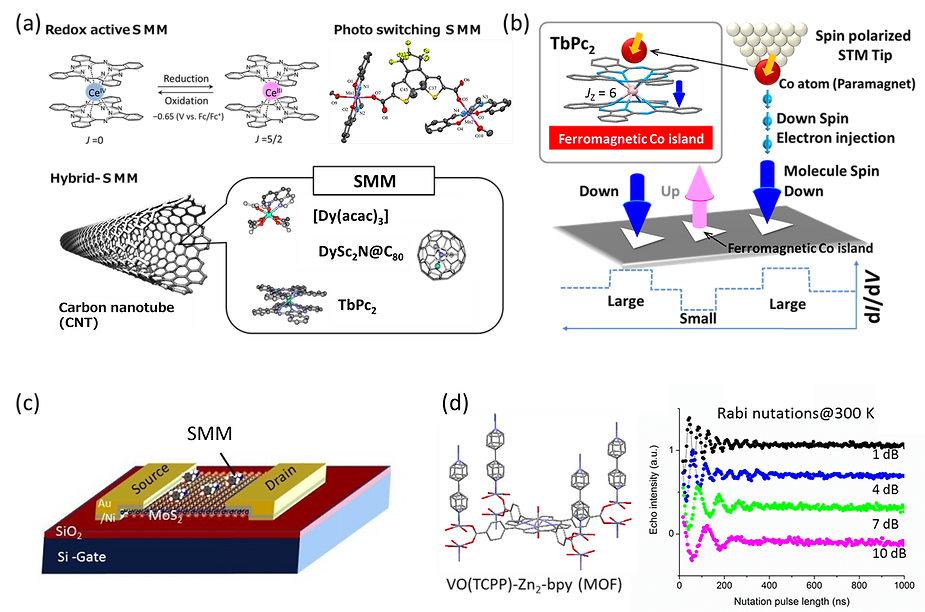
Fig 3. (a) We aim to realize switching using nonmagnetic (CeIV: J = 0)↔SMM (CeIII: J = 5/2) conversion accompanying the reversible oxidation-reduction of cerium. We clarify the current magnetic characteristics of the CNT-containing SMMs. (b) Image of magnetization of SMM (TbPc2) using spin-polarized tunnel current with atomic-scale localization. (c) Create a MoS2 single layer FET device, place TbPc2 (SMM) on the MoS2 part, and aim for FET control by using the spin direction of TbPc2. (d) We have increased the coherence time of the molecular spin by making 0-dimensional vanadyl-porphyrine [VO(TCPP)], a three-dimensional MOF (molecular organic framework) structure. We clarify the correlation between spin coherence time and lattice dimensionality (0 to 3 dimensions).
(4) Importance and Necessity of this Project and its Expected Impact on Broader Research Fields
First, as far as single-molecule nanospintronics is concerned, the most important thing is to manipulate and control the spin current. In recent years, organic molecules can maintain their spin currents for a long time (or distance) as compared with metals, and so, attention has been focused on molecular spintronics. However, since spin-orbit interactions needed for efficient control is very small, it is difficult to inject spin with uniform orientation in organic molecular materials. On the other hand, rare earth (f electron) SMMs or molecular nanomagnets that the applicant is particularly interested in has spin-orbit interaction. Thus, it is possible to convert between the magnetization/current signal and spin current. However, there have been no reports on spin injection into SMMs so far. By using the method proposed by applicants, spin from a ferromagnetic electrode can be injected into SMMs (Fig. 3b). We think that the method of detecting reversal of the SMM magnetization direction using this spin-polarized current greatly affects the future of spinterface research fields. And addressing and reading of single spins in these nano-molecular devices can be demonstrated experimentally.
Second, since f electrons are shielded by d electrons, it has been thought that correlation with π electrons is not possible. In other words, this problem has not been studied academically. Therefore, by obtaining knowledge about the π-f interactions between the spin of f-based SMMs and conduction carriers and by controlling FET characteristics using f-based SMMs, the boundaries of this research can be expanded to the bulk state (crystal/thin film). Thus, spin injection into SMMs becomes possible.
Third, molecular magnetic materials can be used as spin qubits enables quantum devices based on spin states. By utilizing self-assembly of molecules, it is possible to control the time that the qubit is kept, so quantum computation becomes possible, bringing science closer to the realization of quantum computers.
By carrying out this research, scientific and technical problems can be overcome by using SMMs and molecular nanomagnets. We are uniquely positioned to overcome problems in a limited period and can establish a strong foundation from fundamental research to applications of spintronics using SMMs.
(5) Research Achievements of the Applicant(s) Relevant to this Project
Prior to this task, we succeeded for the first time in observing Kondo resonance switching due to the reversible structural change of TbPc2 molecule adsorbed on an Au(111) substrate (Komeda & Yamashita, Nat. Commun., 2011). Studies on single-molecule spins were found to be strongly influenced by the electronic state of the magnetic molecules when in contact with metal electrodes (substrates). Nevertheless, progress in studies on interfaces formed by molecules and single molecules assembled on various substrate surfaces are important as a starting point for studying single SMM properties. In addition, we developed a new technology to detect the reversal of the magnetization direction of the double Co film (island structure) by using a spin-polarized current.
Project 2.
Innovative Functions Originating from the Unexploited Electronic States in Nanowire MX Metal Complexes
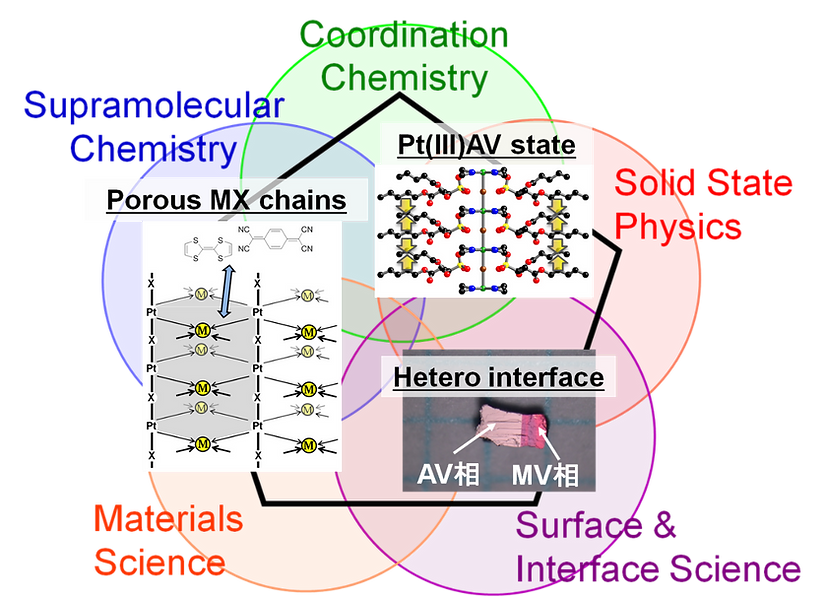
【Purpose and Background of the Research】
The Discovery of new electronic states in solids often develops new functions and research fields. For instance, conductive organic polymers, superconducting copper oxides, carbon nanotubes, and graphene have been widely investigated because of their unique electronic states. Thus, it is important to develop the materials which have the multistability of electronic states.
One-dimensional (1D) electron system intrinsically provides various electronic states and characteristic electronic properties based on the strong correlation between electrons and lattice. Therefore, the 1D electron system is key to create a platform for exploring new electronic states and properties. In this research, we especially focus on quasi-1D halogen-bridged metal complexes (MX chains), which have high tunability and rich electronic properties derived from organic and inorganic components, respectively. We believe that the development of new electronic states in MX chains will give us unprecedented innovative electronic functions.
【Research Methods】
(1)Creation of innovative electronic functions based on Pt(III) averaged valence state
MX chains have the bistability of electronic states (i.e. M(III) averaged valence (AV) state and M(II/IV) mixed-valence (MV) state). Although Pt complexes are most promising for achieving the high functionalities, all Pt complexes reported so far form MV state. In this subject, the techniques which successfully stabilized AV state in Pd complexes will be applied to Pt complexes (e.g. attractive force between alkyl chains and multiple hydrogen-bond networks).
(2) Development of new electronic structures in nano and hetero interface (p-n junction)
Heterojunction crystals and superstructures will be fabricated by epitaxial crystal growth from solution by using electrocrystallization. The electronic structure around the interface will be detected by STM and Raman spectroscopy. Moreover, we fabricate the heterojunction of p-type and n-type semiconducting MX chains to study the characteristics of the single-crystalline device.
(3) Modulating band filling by chemical doping in porous MX chains
In contrast to inorganic materials, it is quite difficult to achieve chemical doping in molecular crystals by ion substitution or defect, because the molecules have a certain size. To overcome this problem, we introduce porosity to MX chains and conduct carrier injection by the redox reaction with guest molecules.
【Expected Research Achievements and Scientific Significance】
In addition to the enhancement of gigantic third-order nonlinear optical susceptibility, various novel properties such as metallic conduction, diode characteristic, molecular-responsible switching will be developed. Moreover, the control of band filling should enhance the understanding of molecule-based materials with a 1D electron system.
【Publications Relevant to the Project】
1)H. Kishida, H. Matsuzaki, H. Okamoto, T. Manabe, M. Yamashita, Y. Taguchi, and Y.
Tokura, Gigantic Optical Nonlinearity in One-Dimensional Mott-Hubbard Insulators,
Nature, 405, 929-932(2000)
2)M. R. Mian, H. Iguchi, S. Takaishi, M. Yamashita et al., Multiple-Hydrogen-Bond Approach to Uncommon Pd(III) Oxidation State: A Pd−Br Chain with High Conductivity and Thermal Stability. J. Am. Chem. Soc. 139, 6562–6565 (2017).